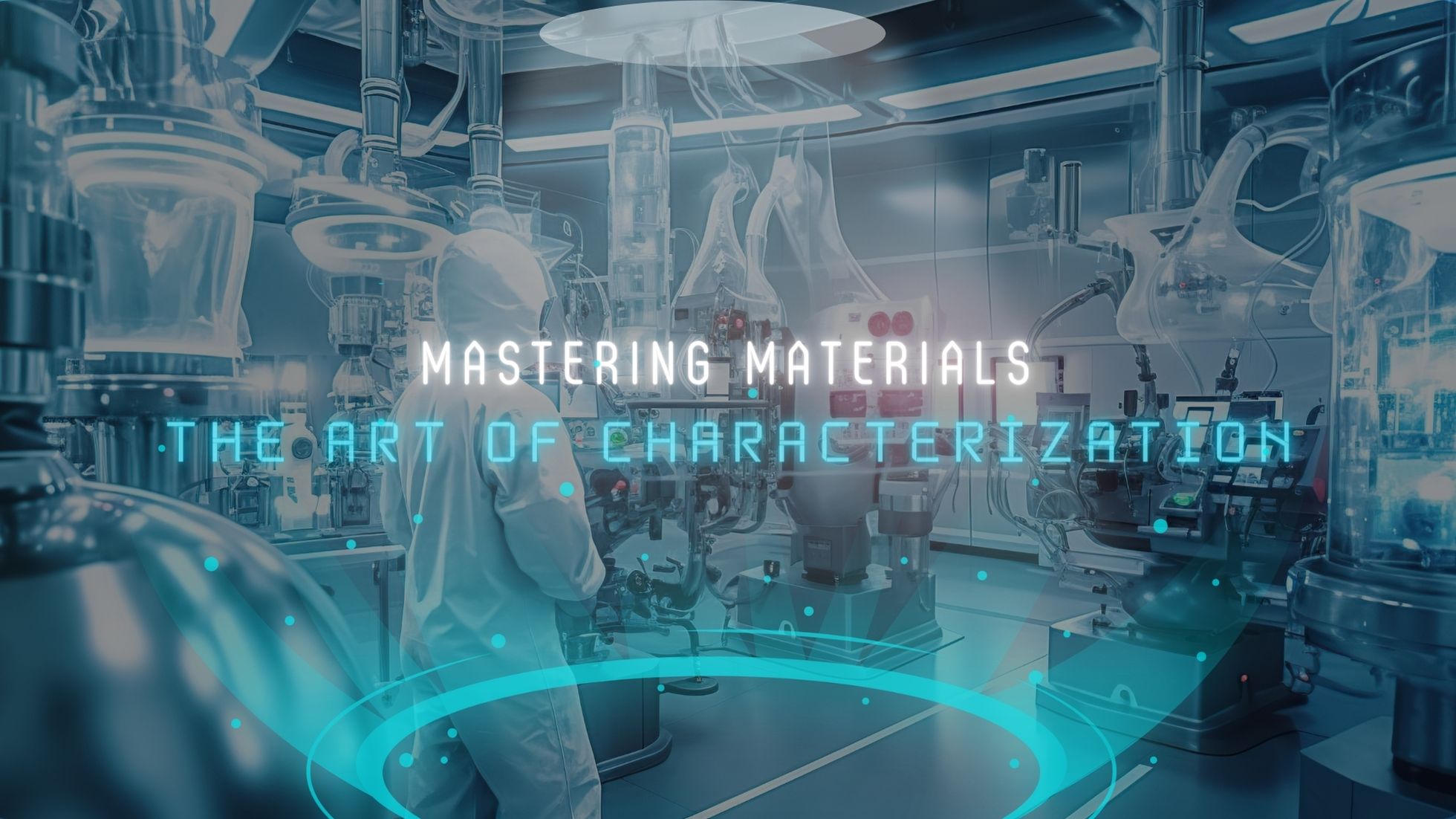
The Art of Characterization
Mastering Materials
Characterization serves as the cornerstone of materials science, influencing various aspects of scientific research, industry, and technological progress. This chapter delves into the fundamental importance of characterization and its multiple dimensions.
Uncovering the Nature of Materials
At its core, the primary objective of materials characterization is to unravel the intrinsic nature of various substances. This includes understanding their physical, chemical, and structural properties. Without this understanding, materials would remain enigmatic entities, hindering innovation and practical applications.
Consider a piece of steel, for instance. Through careful characterization, we can determine not only its composition but also its crystalline structure, which affects properties such as strength and ductility. This knowledge is the starting point for designing materials to meet specific needs.
The Foundation of Materials Science
Materials science, as a discipline, relies heavily on characterization. It provides the scientific basis for understanding, designing, and developing new materials. Materials scientists use characterization to explore the properties of both natural and synthetic materials, enabling them to engineer materials with tailored properties for various applications. For example, in the development of advanced ceramics for use in aerospace components, characterization techniques help identify the ideal combination of elements and crystalline structures to achieve the desired strength-to-weight ratio and high-temperature stability.
Quality Assurance and Control
In the industrial and manufacturing sectors, quality assurance is paramount. Characterization plays a pivotal role in quality control. By meticulously assessing the properties of raw materials and finished products, manufacturers ensure consistency and adhere to regulatory standards. This quality control ensures that the products are not only safe but also reliable.
A classic example is the pharmaceutical industry. Here, characterization techniques help confirm the identity and purity of active pharmaceutical ingredients, ensuring that every pill or injection adheres to the highest standards of safety and efficacy.
Troubleshooting and Problem Resolution
When materials or products exhibit unexpected behavior or fail to meet performance expectations, characterization becomes the beacon of clarity. It enables scientists and engineers to diagnose and solve problems efficiently. By examining the properties, composition, and structure of materials, they can pinpoint the root cause of the issue.
For instance, in the electronics industry, where even minute defects can lead to catastrophic malfunctions, characterization helps identify issues like solder joint failures, corrosion, or material impurities, leading to more reliable and robust electronic devices.
Advancing Technology
Characterization is the driving force behind technological advancements. It empowers the development of materials with precisely tuned properties. Advanced polymers, high-performance alloys, semiconductor materials, and nanomaterials are the products of meticulous characterization.
These materials underpin innovations in electronics, healthcare, renewable energy, and more. The development of new materials, informed by characterization, continues to shape our world in profound ways. From smartphones to medical implants to clean energy solutions, these technologies owe their existence to the careful and systematic understanding of materials.
Modern Advancements in Characterization
The field of materials characterization is continually evolving, with new techniques and approaches expanding our understanding of the material world.
Nanomaterial Characterization
As nanotechnology continues to advance, the characterization of nanomaterials has become increasingly critical. Specialized techniques, like Atomic Force Microscopy (AFM) and Transmission Electron Microscopy (TEM), have emerged. These methods provide unprecedented insights into nanoscale structures and materials.
In fields such as electronics, materials science, and healthcare, nanomaterials are transforming the landscape. Their unique properties are harnessed to create novel devices, sensors, and drug delivery systems. Advanced characterization methods enable us to understand and harness these materials effectively.
Atomic Force Microscopy (AFM): AFM is a powerful technique that allows researchers to image and manipulate nanoscale structures with incredible precision. By scanning a sharp tip over the sample’s surface and measuring the interactions between the tip and the sample, AFM provides topographical information at the atomic scale.
Transmission Electron Microscopy (TEM): TEM is a high-resolution imaging technique that uses a focused beam of electrons transmitted through an ultra-thin specimen. It can provide atomic-level details of nanomaterials, revealing their structure and composition with unparalleled clarity.
In-Situ Characterization
The field of in-situ characterization is growing in importance. In-situ characterization involves studying materials under real-world conditions, whether it’s extreme temperatures, high pressures, or reactive environments. This approach is crucial for understanding dynamic processes, such as chemical reactions, corrosion, and phase changes.
The data acquired through in-situ characterization is invaluable for designing materials that perform optimally in specific conditions. Whether it’s developing materials for aerospace applications or improving catalysts for industrial processes, in-situ characterization provides a deeper understanding of how materials behave in practical scenarios.
High-Temperature Characterization: In-situ characterization at elevated temperatures is vital for materials used in extreme conditions, such as turbine blades in jet engines or materials in spacecraft. Researchers can study how materials react and change under high heat, enabling the development of heat-resistant and high-performance materials.
Corrosion Studies: Understanding how materials corrode in real-world conditions is critical for infrastructure, transportation, and marine applications. In-situ characterization allows scientists to observe corrosion processes as they happen, leading to the development of more durable materials and coatings.
Computational Characterization
Advancements in computing have given rise to computational methods for characterizing materials. Molecular dynamics simulations and quantum chemistry calculations allow researchers to predict material behavior, screen for new materials, and design tailored molecules with desired properties.
Molecular Dynamics Simulations: These simulations use the laws of classical mechanics to model the motion of atoms and molecules over time. They provide insights into the behavior of materials at the atomic scale, helping researchers understand properties such as mechanical strength and thermal conductivity.
Quantum Chemistry Calculations: Quantum chemistry uses the principles of quantum mechanics to model the behavior of electrons in atoms and molecules. It is particularly valuable for understanding chemical reactions, electronic properties, and the structure of complex molecules.
Computational characterization is particularly valuable in the early stages of material development. It enables researchers to explore a vast design space, saving time and resources by identifying the most promising candidates for experimental testing. In fields like drug discovery, where developing new molecules can be time-consuming and costly, computational characterization is a game-changer.
The significance of characterization extends far beyond its role as a scientific tool. It is the bedrock of materials science, quality assurance, troubleshooting, and technological advancement. With modern advancements in characterization, we continue to push the boundaries of scientific understanding and drive innovations that reshape our world.